Areas of Research
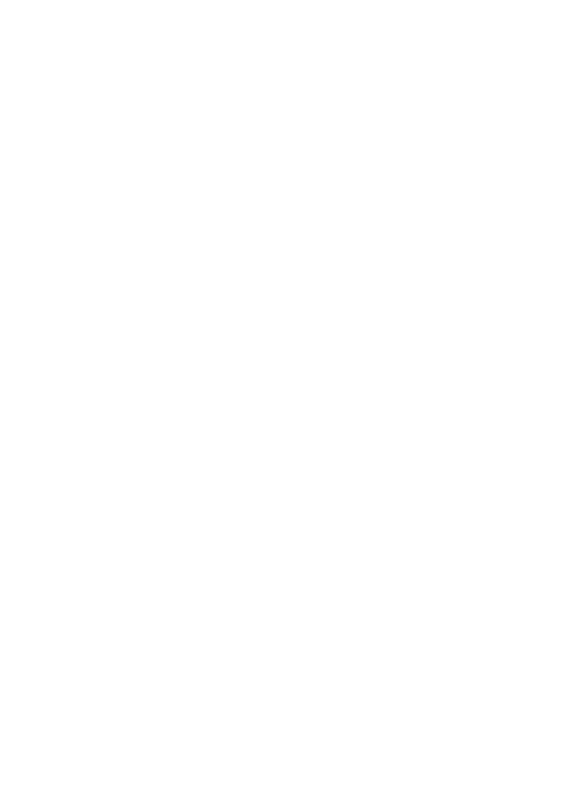
Our Researchers
Our People
Our people are at the heart of what we do. We recognise that excellent research develops from both the distinctive work of individuals as well as collaborative efforts. Find out more about our people here.
Postgraduate Vacancies
Interested in pursuing a postgraduate path with Tyndall? Discover more about our postgraduate scholarship opportunities here.
Join Us
Big things are happening here at Tyndall. If you want to make a difference to the world around you and work with some of the best minds in research – come and join us!
Latest Research News
Get the latest research news on our prestigious awards, game changing technologies, researcher spotlights, exciting initiatives and more.