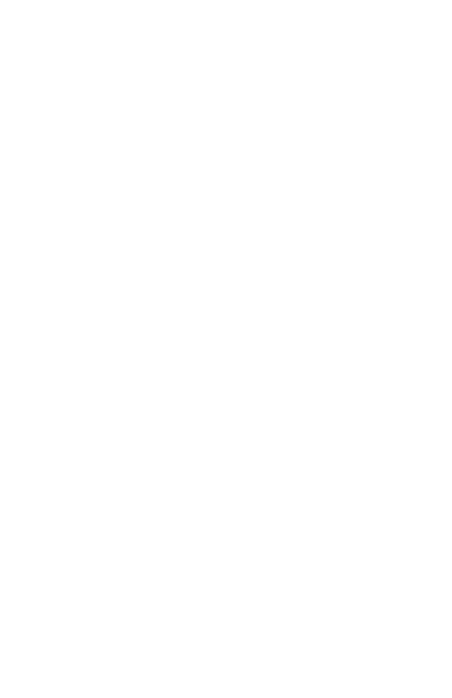
Biophotonics
The Biophotonics research programme was established to conduct research and develop novel Biophotonics techniques, especially towards integrating technologies, research teams, sectors and companies to deliver novel, smart surgical tools and diagnostic systems into the hands of clinicians.
Our mission within Biophotonics at Tyndall is to transition techniques into miniaturised form factors designed for clinical deployment and for manufacture, ensuring that this ambitious research programme also delivers commercial deployment and societal impact. This will result in a steady flow of major novel technology innovations from the Biophotonics labs and into the clinical setting. These activities are well aligned with Tyndall’s and Ireland’s strategic interests, being hot topics in two identified priority areas of research – Photonics and Medical Devices.
Research Challenge
In the future, surgeons will require the ability to generate high quality, diagnostic images deep within the body using micro-scale instrumentation such as arterial guidewires.
The programme’s vision is therefore working towards developing the world’s smallest integrated imaging system for guided surgery.
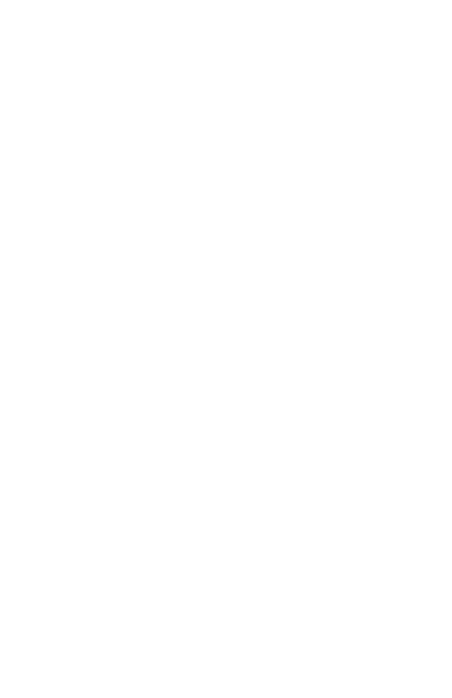
Biophotonics Technology Solutions
Acousto Optical Tomography (AOT) is a multimodal imaging technique that aims at obtaining optical information from deep within biological tissue by using ultrasound and light. It has huge potential for imaging deep into biological tissue. The Biophotonics team are combining AOT techniques with heterodyne holography and wavefront shaping to establish new methods that can help propel AOT in the clinical realm.
In AOT, a laser illuminates a medium, while an ultrasound transducer sends short ultrasound pulses. Some of the light that travels through the ultrasound focused pulse within the medium becomes modulated by the ultrasound. This modulated light is called the tagged light. By using advanced detection methods, the tagged light signal can be distinguished from the background laser light and information about the optical properties at the location of the ultrasound focus can be recovered. This location is known because ultrasound is not scattered in tissue, as a result, it is possible to generate an image point by point. by moving the ultrasound focus within the medium.
Endoscopy research within Biophotonics is focused on developing the next-generation chip on tip surgical endoscopes for surgical guidance and automated robotic surgery. Endoscopy plays a pivotal role by enabling surgeons to access and visualize intricate anatomical structures. The modern manual/robotic surgery requires innovative technologies to enhance precision, minimize invasiveness, to improve patient outcomes. The efficacy of endoscopic interventions is intrinsically linked to the quality of imaging and size of endoscopes. The team aims to push boundaries and create new frontiers with the novel chip on tip platform.
The key challenges addressed are:
- Smallest: a small footprint (< 3 Fr)
- Fastest: a higher frame rate to reduce motion artefacts
- Accurate: hyperspectral imaging for multi-biomarker quantification.
To achieve these goals, the multidisciplinary core team are developing all-optical, novel communication and powering platforms which are tailored to enable chip-on-tip endoscopes to probe deep within the body.
Recent Publications
M.S Nogueira, S. Maryam, M. Amissah, S. Killeen, M. O’Riordain, S. Andersson-Engels,
”Diffuse reflectance spectroscopy for colorectal cancer surgical guidance: towards real-time tissue characterization and new biomarkers”, Analyst, 149, 88-99 (2024).
S. Konugolu-Venkata-Sekar, H. Ma, K. Komolibus, G. Dumlupinar, M.J. Mickert, K. Krawczyk, S. Andersson-Engels,
“High contrast breast cancer biomarker semi-quantification and immunohistochemistry imaging using upconverting nanoparticles“, Biomedical Optics Express 15, 900-909 (2024).
S. Matias, K. Komolibus, K. W. Kho, S. Konugolu-Venkata-Sekar, S. Andersson-Engels,
“Generalised analytical model of the transition power densities of the upconversion luminescence and quantum yield”, Nanoscale Advances, 5, 3279-3286 (2023).
Project pHetalSafe
The project pHetalSafe aims to develop a cutting-edge fetal sensor designed to detect and prevent fetal hypoxia, which is insufficient supply of oxygen to the brain during birth. This critical condition affects nearly 200 newborns in Ireland annually and tragically leads to death or lifelong disability for over one million infants worldwide each year. Moreover, it can cause severe brain damage and permanent neurological impairments in newborns.
The proposed and innovative fetal monitoring system is a minimally invasive, multimodal solution that applies novel optical technology to provide real-time assessment of lactate and pH levels during labour. Other vital physiological parameters such as fetal heart rate and temperature can potentially also be measured.
The project has been awarded €1.35m in funding from Enterprise Ireland for Tyndall and its collaborators at the INFANT Research Centre in UCC, together with the Cork University Maternity Hospital. This ambitious research and commercialisation program will accelerate the development of the fetal sensor with a view to bringing it to market.
Project MedPhab
The purpose of the MedPhab pilot line is to accelerate photonic device development for medical applications with the intention of helping customers to fast-track their “idea to product” pathway. MedPhab was formed as a united consortium of partners from leading research centres and industrial players in medical devices and photonics.
MedPhab provides a versatile technology platform for companies to create solutions across a range of applications. These include real-time monitoring of treatment progression in hospital environments, medical wearables and equipment for in-vitro diagnostics. MedPhab has established comprehensive systems, procedures and protocols that are adaptable to all industry partners and RTOs. These resources are designed to assist in navigating the process of device design, standardisation and manufacturing.
MedPhab is a sub-sequent of the MedPhab pilot line EU project under Horizon 2020 research and innovation programme No. 871345. Find out more https://medphab.com/.
Research making a difference - Biophotonics Researcher Dr Shree Krishnamoorthy
Dr Shree Krishnamoorthy shares her inspiring career story and a snapshot of her research with the Biophotonics team at Tyndall.
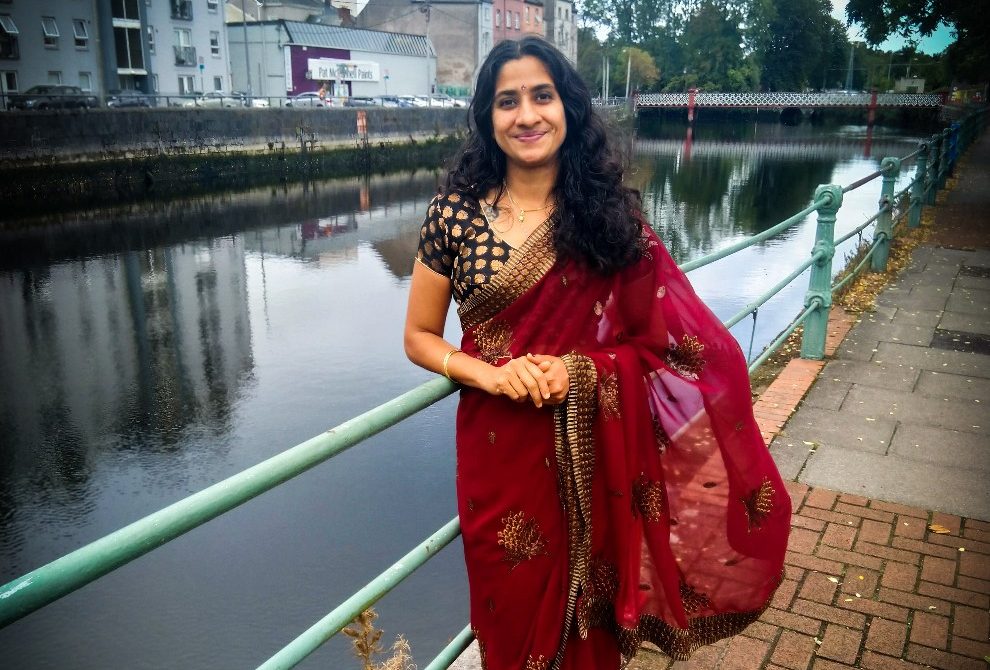